04 May 2018
Superconductor research published in PRX
Dr Cedric Weber, Department of Physics, has been published in the Physical Review X, the journal of the American Physical Society for a paper detailing research carried out in the department to unravel how to get superconductors to work at higher temperatures. Currently, superconductors only work at low temperatures: -100 kelvin or -170c, and it takes a lot of energy to cool them to these temperatures.
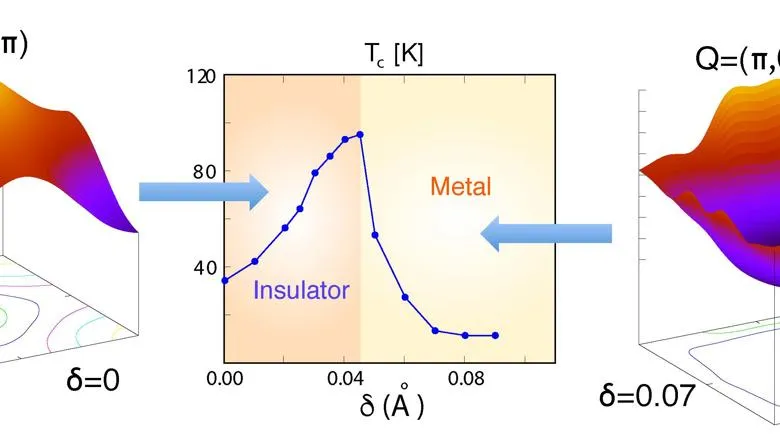
Superconductors produce a strong magnetic field and have less resistance when an electric current is passed through them. Regular conductors lose approx. 30% of the electrical energy flowing through them as heat. Natural magnetic materials typically produce a magnetic field of only a few Tesla (the unit used to measure magnetic field strength) so more powerful magnetic fields, used in MRI scanners and MagLev trains, require superconductors.
Under normal conditions electrons repel each other; this is known as the Coulomb force. However, quantum theory shows us that in special conditions and at low temperatures this force can be overcome allowing electrons to bind into pairs called Cooper pairs. As the electrons are not repelling each other, they do not dissipate and carry their charge without losing electrons along the way as heat. This is called superconductivity. There are two types of superconductivity: conventional and unconventional. Conventional superconductivity is well understood and occurs when the Cooper pairs also bond with phonons. Unconventional superconductivity is less well understood and has many causes other than phonons. It is hoped that this unconventional type may allow for superconductivity at room temperature.
Since its discovery in the mid 1980's, the quest for unconventional superconductors working at a higher temperature, based on oxides of copper, have challenged and pushed the frontiers of theoretical and experimental abilities. Finding a unified description or single tuning parameter that drives a superconductor towards higher critical temperatures continues to be a key but elusive question in this field. Most efforts to achieve a room temperature superconductor without adding more energy to excite the electrons have failed. However, recently it has been achieved for short timescales (measured in picoseconds) when a laser excites the electrons by providing energy into the system.
Within the paradigm of a newly developed starting theory, which incorporates both local and non-local correlations, Dr Cedric Weber and his team, have shown that the copper-oxygen bond length can be used as a unique tuning parameter driving a copper oxide between a Mott insulator and a metal (metal-insulator transition) with an accompanying maximum temperature for the superconductor. It was found that the critical temperature for the superconductor continues to increase when the electrons were arranged in a checkboard pattern (known as staggered magnetic order vector) and were at their maximum fluctuation. However, both the temperature and electron fluctuations dropped significantly when the electrons were arranged in a stripe pattern. With this knowledge, and how these patterns can be manipulated using the length of the copper-oxygen bonds, a greater understanding of how to optimise the critical temperature of a superconductor can be reached.
With this new theoretical breakthrough, the team have been able to connect the dots linking small changes in the very small distances between electrons to dramatic changes in the critical superconducting temperature in cuprates (materials containing copper). The researchers believe it has the ability to push this field towards new frontiers in experimental and theoretical understanding of enhancement of temperature in unconventional superconductors by providing a guide on how to control their critical temperatures. Furthermore, Tetrahertz spectroscopy could be used to determine the amount of energy needed to excite the electrons in materials enough to distort the copper-oxygen bonds and raise the critical temperature required for the superconductor to work. It may also lead to discovery of whether other materials can be manipulated in this way to act as superconductors.
These developments could eventually lead to smaller wind turbines (as power output is dependent on a magnet) and use in quantum computing and nano-technology.