11 June 2019
Mathematics researcher experimentally confirms the behaviour of one-dimensional gases of ultra-cold atoms for the first time
Dr Benjamin Doyon, Department of Mathematics, and collaborators have for the first time observed how very cold clouds of atoms behave when they are made to only travel in one direction. This confirms the generalised hydrodynamics theory Dr Doyon co-developed in 2016 on how these ultra-cold atomic gases constrained to one dimension would behave.
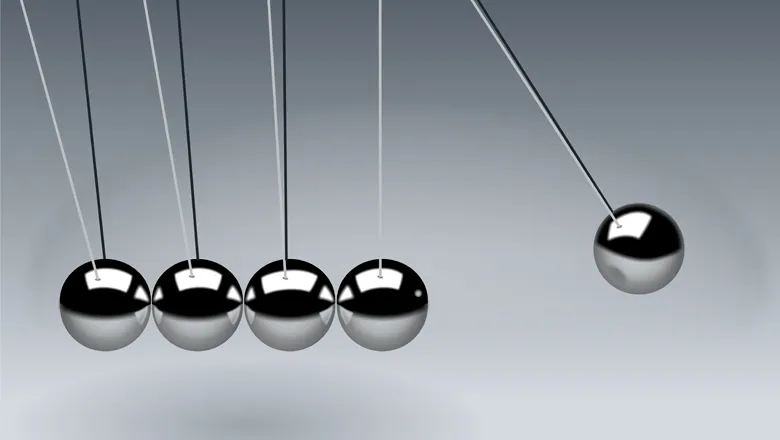
Dr Benjamin Doyon, Department of Mathematics, and collaborators have for the first time observed how very cold clouds of atoms behave when they are made to only travel in one direction. This confirms the generalised hydrodynamics theory Dr Doyon co-developed in 2016 on how these ultra-cold atomic gases constrained to one dimension would behave.
Hydrodynamics is a very old theory that describes many familiar elements of everyday life, such as water and air. Its equations are simple enough to be simulated on a computer, and it gives a lot of insight into the wide range of behaviours shown by fluids. Water, air and other fluids are made up of lots of particles which all interact with each other and so mathematically describing how they all move and interact with each other, is very difficult.
This is especially true in the quantum world, where simulating the probability of how lots of particles in a system will behave requires exponentially large quantities of computing resource. By contrast, hydrodynamics is not based on working out the equations for each individual particle and how it behaves, but instead is based on a simple assumption: that in a small ‘cell’ of fluid, many of the particles will have reached, or will be near to, equilibrium; that is that their energy is balanced with those particles around them. In ordinary fluids, hydrodynamic equations explain how quantities of local mass, momentum and energy densities change in space time. The local thermodynamic equilibrium assumption supposes that although there are variations in temperature through a substance, the changes are so slow that equilibrium can be assumed for some point in the material at any given time.
However, the famous quantum Newton cradle experiment in 2006 showed that these simple hydrodynamic equations unexpectedly fail in some situations. This experiment cooled clouds of gas atoms and trapped them into ‘tubes’ of lasers so that they could only move in one direction, and therefore only move one dimensionally. A laser was then used to propel half the atoms in one direction, and half in the other, and then back together. With the theory of hydrodynamics it was expected the two clouds would hit each other and start to balance their energy, through temperature and movement, until they reached equilibrium. However, the two clouds continued to move through each other and back like a pendulum.
The world of quantum one-dimensional physics is particularly rich and complex, and in many cases very different from the three-dimensional world. Indeed, ultra-cold one-dimensional atomic gases seem to show very different properties, as shown in the 2006 experiment. For many years, their hydrodynamic equations were not known, and as a consequence there was no simple theory able to account for the findings of the quantum Newton cradle experiment, or growing number of experiments probing ultra-cold atomic gases in low dimensions.
In 2016, two teams, one led by Dr Doyon, devised a theory, using different methods, which laid the basis for the hydrodynamic equations of interacting one-dimensional gases. The theory is extremely general, and applies to a wealth of one-dimensional systems, quantum and classical. It is based on the property of integrability, whereby a system displays an extensive number of conservation laws, for example, the total amount of energy in a system does not change over time. These conservation laws modify the hydrodynamic equations in fundamental ways, leading to what was dubbed ‘generalised hydrodynamics’. Ultra-cold atomic gases fall within the category of one-dimensional systems that are integrable, and so obey generalised hydrodynamics. Their basic equations were first described by Dr Doyon’s team in its 2016 paper, and the full theory able to describe quantum traps, where electrical or magnetic fields are used to trap atoms, such as those used in the quantum Newton cradle, was formed by Dr Doyon and his student in 2017.
This mathematical theory was confirmed in an experiment carried out by Dr Doyon’s team which observed the behaviour of super-cooled atoms. The team used magnetic fields to trap super-cooled gas atoms in one dimension and by changing the force of the field, made them move. They were then able to observe and image how the atoms behaved, which confirmed the mathematical hypothesis.
Having workable hydrodynamic equations now opens the way for the discovery and understanding of many of the strange properties of one-dimensional quantum systems, with potential applications to quantum wires, which can be used to make more efficient transistors, and next-generation logical circuits for use in smaller, more efficient electronics.
The discovery of generalised hydrodynamics has sparked a large amount of theoretical activity since 2016 and it is now starting to reach the experimental realm. But it does not stop there: the theory is flexible enough to describe a large family of models, both quantum and classical, be they chains of atoms in interactions, field theories, or even cellular automata, a type of mathematical model where individual parts, and therefore the whole, change over time according to specified rules.
The implications are just starting to be explored.
This research has been published in the American Physical Society journal Phys. Rev. Lett. and in Physics Today.